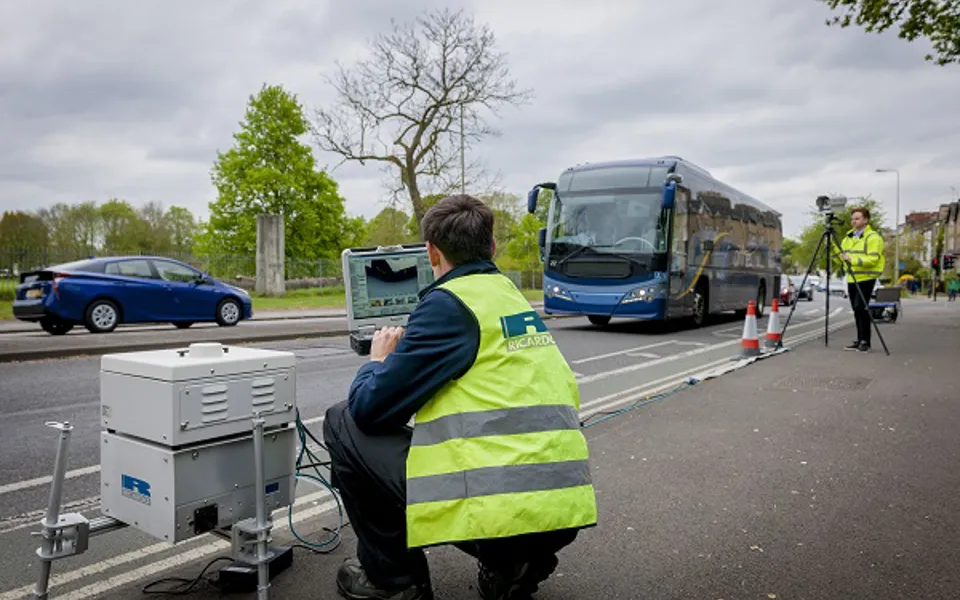
Failed remote sensing measurements provide insights into hybrid vehicle behaviour
15 Dec 2020
The problem of measuring hybrids
Vehicle remote sensing measures exhaust emissions by absorption spectroscopy, detecting the carbon dioxide (CO2) in a vehicle’s exhaust plume as well as providing a measurement of several air quality pollutants such as nitrogen oxides (NOx) and carbon monoxide (CO). However, measuring hybrid vehicles poses a problem because these vehicles have zero exhaust emissions for the period they are operating using batteries. In other words, remote sensing will ‘fail’ to measure anything. However, it turns out that this failure can provide some interesting new insights into the behaviour of hybrid vehicles.
Understanding the behaviour of Plug-in Hybrid Vehicles (PHEVs) is more challenging than understanding the behaviour of ordinary hybrid electric vehicles because their emissions not only depend on real driving operation, but also on the extent to which the vehicle has been charged in the first place. This human behaviour element makes it even more difficult to establish real driving emissions because it is difficult to define a real-world drive cycle that also captures the how diligent someone is in charging their vehicle. The emissions from these vehicles will also depend on battery size and trip length – longer trips would tend to use an increasing amount of the engine. For these and other reasons, there is a lot of uncertainty over the actual, real driving emissions of hybrid vehicles.
The International Council on Clean Transportation (ICCT) has recently conducted an extensive analysis on the real-world CO2 emissions (fuel economy) performance of PHEVs (Plötz et al., 2020). The ICCT report highlights the potentially large discrepancy between the fuel economy of PHEV vehicles under real driving conditions compared to that achieved under Type Approval test cycles, finding that tailpipe CO2 emissions can be two to four times that reported under regulated cycles. The ICCT use extensive fuel economy data to infer a Utility Factor (UF), which is a measure of the proportion of km driven using batteries. It was found that over the regulated NEDC test cycle, 69% of the driving was using batteries while under real driving conditions the proportion dropped to 37%. It should be noted however that the ICCT estimated the UF for European data sets from reported fuel economy, rather than using measured values of the UF, which are generally not available.
An interesting question is whether it is possible to use vehicle emission remote sensing measurements to calculate a direct measure of the amount of time vehicles spend using batteries under real driving conditions?
Delving into the data
Data in the Ricardo real driving emissions database (https://ee.ricardo.com/transport/vehicle-emissions-monitoring) suggests that 1.6% of conventional petrol vehicles detected fail to result in a valid CO2 plume. This failure can arise for several reasons including only measuring a small part of the dispersing plume, for example. On the other hand, the database reveals that there is a 27% failure rate for hybrid vehicles, which include conventional hybrids, PHEVs and range-extended electric vehicles (REEV).
A REEV is a battery electric vehicle that runs on electricity but includes an auxiliary power unit known as a ‘range extender’, which is usually a small petrol engine. In these vehicles the engine drives an electric generator which charges a battery that supplies the vehicle’s electric motor rather than driving the wheels directly.
Overall, failed CO2 plume measurements are seen for 23.6% of hybrid electric vehicles, 41.2% for PHEV and 91.1% of REEV. These data can be taken as estimates of the amount of time these types of vehicles operate in battery mode; the Utility Factor (UF).
The data also show strong dependencies on other variables such as the power demand of a vehicle at the time it is measured. Figure 1 shows the estimate of the percentage battery use against vehicle specific power (vehicle power demand in Watts divided by the vehicle mass). This Figure shows very clearly that for hybrid electric vehicles that at low power demands the UF is high but drops sharply as the power demand increases. For PHEV vehicles there is a very different relationship that indicates that these vehicles use their batteries over a wider set of conditions. Interestingly, for both vehicle types, battery use seems to increase under much higher vehicle power demands, which might reflect the increased need for battery use to compensate for the lower-powered engines used in hybrids.
Figure 1: Relationship between vehicle power and percentage of time on batteries for electric hybrids and PHEV vehicles. The size of the circles shows the number of measurements.
There is a similar relationship for vehicle speed, shown in Figure 2. The relationship is not as strong as Figure 1, which reflects the inherently stronger relationship with vehicle power than vehicle speed. Nevertheless, Figure 2 does indicate that at lower speeds, the UF tends to increase. Furthermore, a difference is still seen between hybrid electric vehicles and PHEVs. Although not shown, REEVs showed an average 91% time spent using batteries, albeit with a more limited sample of data.
Figure 2: Relationship between vehicle speed and percentage of time on batteries for electric hybrids and PHEV vehicles. The size of the circles shows the number of measurements.
Battery use for urban driving conditions
Remote sensing measurements tend to be made under higher vehicle power conditions than experienced for average urban driving – in part because remote sensing does not measure idling vehicles. However, the information shown in Figure 1 can be used to predict battery usage over urban driving conditions by applying the findings above to lots of PEMS drive cycles (Davison et al, 2020). In this case we have used 58 PEMS tests and over 4,000 km of driving from DfT measurements to calculate the expected battery usage under urban driving conditions.
The distribution of vehicle power for these PEMS measurements is shown in Figure 3. These predictions suggest that under urban driving conditions, hybrid electric vehicles use their batteries for 34.6% of the time and PHEVs 42.7% of the time. The value of almost 43% of PHEVs is similar to the ICCT value of 37% but it might be expected that for urban driving conditions the UF value would be higher. These results may be indicative of people not charging their PHEVs often enough – or indeed choosing one of the driver-selectable vehicle operating modes that blends battery/motor and engine use. While not the focus of this blog, PHEVs extend their advantage over hybrid electric vehicles in rural and motorway driving conditions.
Figure 3: Distribution of vehicle powers observed in DfT PEMS tests considered in Davison et al. (2020).
We started to investigate these effects with a view to correcting the NOx emissions (and other air quality pollutants) calculated for hybrid vehicles i.e., to adjust the measured emissions when using an engine to account for zero exhaust emissions when using batteries. However, these data sets provide a considerable amount of other useful information – even for failed measurements! It is also an issue that can be investigated further by looking at different vehicle manufacturers, different urban areas and the effect of factors such as how hilly a region is.
Acknowledgements
Thanks to Jack Davison, a Ricardo CASE-funded PhD student at the University of York for processing the PEMS data.
References
Davison, J., Bernard, Y., Borken-Kleefeld, J., Farren, N. J. Hausberger, S., Sjodin, A., Tate, J. E., Vaughan, A. R., Carslaw, D. C. (2020). Calculation of Distance-Based Emission Factors from Vehicle Emission Remote Sensing Measurements. Science of the Total Environment. 739 (2020) 139688.
Patrick Plötz, Cornelius Moll, Georg Bieker, Peter Mock, Yaoming Li (2020). Real-world Usage of Plug-in Hybrid Electric Vehicles, International Council on Clean Transportation, September 2020, https://theicct.org/sites/default/files/publications/PHEV-white%20paper-sept2020-0.pdf